Warning: The text reproduced here is a copy of information published elsewhere. This information has either:
- been published freely on the internet and has been cached here as a precaution against future loss of servers and links, or
- been published historically and very few copies of the original text are still available for research purposes.
It is recommended that you look at the original source given below first, and use this text only if that source is not available to you. It is not intended that any text cached here infringe the copyright of the original author. If any copyright owner wishes their text removed from this site, this can be done by contacting the author.
Document summary:
- Title: FTS - The Fast Tube System: A Revolutionary Public Transport System
- Author: Richard J Earle
- Source: http://www.orielsystems.u-net.com/fts/ and http://www.orielsystems.ltd.uk/fts
- Copyright: Copyright © 1999, Richard J Earle
- Date: 12/12/1999 (v2.01)
The Fast Tube System
Abstract: A technical brief of FTS in public domain. A concept comprising a
revolutionary new mode of transport aimed towards addressing the demands
of public and freight transport in the 21st century.
IPR: The Intellectual Property Rights contained herein are protected by patent
application under the international PCT treaty. The concept, ideas and
intellectual property rights are solely owned by Richard J. Earle.
Table of Contents
1. Introduction
2. Background
3. The Concept: An Overview
4. Operational Theory
5. A Capsule in Transit
6. Performance
7. Advantages
8. Disadvantages
9. Station Design
10. Motive Power Cord Attachment
11. Bends, Inclines & Freewheeling
12. Capsule Routing Mechanics
13. Passenger Comfort and Services
14. City-wide versus Inter-city Application Examples
15. FTS in a Public Context
16. Freight
17. Financial
18. Safety
18.1. Derailment/Tube Damage/Obstruction
18.2. Bringing Capsules to a Halt in an Emergency
18.3. Emergency Escape
18.4. Capsule Strength and Integrity
18.5. Terrorism
18.6. Fire
18.7. Station Behaviour in Systems Failure Mode
19. Maintenance
20. Environmental
21. Road Traffic Comparisons
22. Social/Economic Impact
Appendix A - Concept Validation Calculations
A.1 Capsule Velocity
A.2 Capsule Acceleration
A.3 Mainline Vacuum Tube Capacity
A.4 Station Capacity
A.5 Energy Consumption
1. Introduction
FTS is a concept designed for rapid transportation in an efficient, safe and environmentally sound manner. It has the potential to revolutionise public transport, providing a cost-effective alternative to current modes of transport. FTS offers reductions in consumption of hydrocarbons, carbon dioxide emissions, urban congestion, noise pollution and unpredictable journey times experienced with traditional transport methods.
For the first time public transport will have an alternative that competes for commuter traffic on their primary decision criterion; predictable and shorter journey time.
The environmental impact, cost-savings and infrastructure requirements need to be further explored to position the FTS as an attractive alternative to current transportation systems, however the technology to develop this system is currently available.
Although the FTS is in the concept phase of development, considerable research and design have been undertaken to assess the feasibility of this transportation system. A small, experienced team of professionals has dedicated time and effort in the design and research that supports FTS. The concept now presents an opportunity for the design of a pilot implementation and further market research to assess the appetite for FTS in strategic locations in the developed world. The concept is based upon sound engineering principles and presents an exciting transition to a safer, cleaner, faster and a more efficient system of transport.
This paper outlines the technical aspects of FTS and contains an overview of the significant strengths and opportunities of the concept. An investment package is available upon request, with detailed cost estimates, financing options and expectations for return on investment.
2. Background
The keywords are: fast, environmental, and no waiting/queuing. Even disregarding safety there is a practical limit to the speed at which a car can travel as fuel consumption increases exponentially with speed. This happens because the car must displace it's own volume in air as it travels forward. As speed increases an ever stronger force opposes the car. Only by reducing the drag co-efficient (more aerodynamic) can speed increase without aggravating fuel consumption. Perhaps the most aerodynamic of all cars would be the Thrust world record breaker. Even this car is ultimately limited by its drag co-efficient. At walking speed it can be moved by a few men, but the drag co-efficient dictates that to reach high speeds two enormous engines are needed.
At any one time, each of our major highways takes up to 100 k cars per day, all burning hydrocarbons to displace air. There is much less friction with modern cars and lubricants; unless the car is climbing a hill or accelerating, the vast majority of consumed fuel is used to displace air.
No driver sets out to displace air, it's simply a wasteful and unwanted by-product of the process of motoring. Even the most aerodynamic of cars consumes many litres of fuel to travel relatively short distances at low speeds, whilst a satellite on the other hand, can orbit the earth travelling much faster, much further and consuming no fuel at all. The difference being that one must displace air whilst the other does not. The fuel cost in displacing air cannot be overstated.
3. The Concept: An Overview
In essence, FTS is a system that removes air resistance making the drag co-efficient irrelevant. There is then (theoretically), no speed limitation and no fuel consumed (excluding bearing efficiencies and gradients).
Instead of a road the car will travel within a tube. Unlike a conventional car, the FTS car could more effectively be described as a pressurised capsule on wheels. From idle, the capsule will accelerate to full speed and complete it's journey at maximum speed without interruption or slowing for any reason. Only decelerating in order to come to rest at its ultimate destination. Within a given tube all capsules will travel in the same direction and at the same constant speed to enhance efficiency. The capsules will not provide their own motive power, instead they will obtain it from a common source delivered through the tube itself. The system will be entirely automatic with no 'driver' role.
Ultimately there will be a network of tubes and stations, as there is a rail network today. Tubes will be in pairs to provide for both directions of travel. FTS will be financially efficient. Laying of tubes will be cheaper than road or rail, more comparable with the cost of water or gas mains. The capsule cost will be significantly less than car costs, but station costs will be high as will the initial design and development costs.
4. Operational Theory
With a normal car, speed is determined by the driver, or the speed of impeding vehicles. With FTS the capsule speed is, by design, constant for all capsules. There can be no traffic jams, stop lights, queuing or rubber necking. The capsules contain passenger air supply and life support systems with passengers travelling individually or as couples. The vacuum within the tube will be generated when the system is first commissioned and never released, except in the event of disaster recovery. The vacuum provides speed, efficiency and noise benefits by avoiding air resistance. The capsule rides on it's own wheels using a track within the vacuum tube. Rather than the capsule providing its own motive power, the capsule is driven at a constant high speed by attaching to a moving chain, cord or the like (hereafter known as the motive power cord). This is an essential technical feature as self powered capsules would compromise the requirement for light capsules, all travelling in unison at the same constant speed. The capsules must be light as they need to accelerate very quickly at launch time (and likewise decelerate on completing the journey). Further, individual motive power would be impractical due to the serious consequences if one capsule were to loose power.
The motive power cord runs both in the direction of travel and then back in the opposite direction in a continuous loop. With tubes running in pairs, the motive power cord will generally be a loop travelling up one tube and back down the other. However, it is not necessarily the case that one motive power cord exists running the entire tube length. Passengers depart and arrive at specific stations along the vacuum tube. After leaving the station the capsule, which contains the passenger(s), accelerates in a second launch tube to gain the necessary speed before joining the main vacuum tube. The launch tube is also evacuated and runs parallel and close to the main tube. Launch tubes need only be short, but providing sufficient length to attain the necessary speed. A similar tube for deceleration will be needed in the station approach. Acceleration, deceleration and transfer between the vacuum tube and the acceleration tube will be carefully controlled by an automatic control system, which will time the launch of any capsule to coincide with a suitable gap in traffic.
The patent also provides for stations where acceleration and deceleration takes place within the main tube itself. However, this is not likely to be a common configuration as, although it might reduce costs, it degrades overall system capacity. In such a case, any capsule destined for a station where no launch tube exists must arrive with sufficient tail gap such that deceleration can safely be achieved and the capsule removed from the main track without a collision involving follow on capsules. Such a gap would be maintained by the automatic control system by way of delaying the launch of capsules that would otherwise occupy the required gap.
For every capsule, the automatic control system must have knowledge of departure, destination, transit and arrival times. It must keep continuous record of the position and progress of each. In essence, the automatic control system will plan the entire capsule journey, allocating all necessary time slots, tube, systems and equipment availability in advance. The automatic control system will not launch the capsule until all required resources can be assigned. The role of the automatic control system is fundamental for a number of reasons. For example, without it, more passengers could depart for a given station than the station is able to accept. Capsules have no means to control their own speed and the main tube can handle many more capsules than any single station. When the capsule arrives at the station it is conveyed to and locked against a passenger capsule access door within the station. Both the station and the capsule contain automatic doors, which open together, but only when the capsule is locked in position with the vacuum protected by an air seal. The only place capsules meet common air pressure is at the capsule access door.
Commonly stations will provide queuing of capsules in a storage area such that the station can accept a flow of incoming capsules and, if necessary, stack them up awaiting use of the passenger capsule access doors. The storage area is also a vacuum and also contains empty capsules awaiting new passenger departures. Capsules are manipulated within the storage area using automatic handling mechanics. Provision of a station storage area permits the automatic control system to dispatch more capsules bound for that station. Typically, the automatic control system will only dispatch to a station, as many capsules as that station can safely handle. A capsule storage area significantly increases the number of capsules a station can safely handle.
The automatic control system may also dispatch empty capsules under some circumstances. One reason is to manage the volume of empty capsules in the storage area at each station. The number in storage must not be excessive so as to consume the storage space which otherwise allows for a larger number of capsules, bound for the station, to begin their journey elsewhere. On the other hand, too few empty capsules obviously could restrict the flow of departing passengers. Another reason that the automatic control system might need to dispatch empty capsules is for mechanical considerations, specific to the track and tube. For example, sagging in the motive power cord due to gravity might be a problem which could be addressed by providing a maximum inter-capsule gap. Such a gap will be maintained by the use of empty capsules. Equalising the load around the system might also be a consideration for which empty capsules could be used.
5. A Capsule in Transit
Figure 1 below is intended to illustrate some of the engineering aspects of the system and shows a vacuum tube from which the air has been extracted, containing a particular capsule. The view is a cut out in cross section. Often such a vacuum tube will be one of a pair, providing for both directions of travel. For clarity and simplicity only one tube from the pair is shown. Further, only one passenger is shown within the capsule. It is likely that passengers can travel in pairs or as couples, subject to the design specifics of the implementation.
Although not obvious in cross section, the capsule rides on four wheels providing for movement along the track (rails). The illustration shows only one front and one rear wheel, each of which are in reality one of a pair on a common axle. The rear axle/wheels supports the rear of the capsule etc.
The motive power cord is labelled, with the automatic attachment mechanism transferring motion to the capsule. The life support system provides a constant delivery of oxygen/air for the passenger. The capsule door is on the top of the capsule when horizontal, above the passenger in the drawing, but not shown. When the capsule arrives at the target station it will be re-orientated vertically to allow for the passenger to step out.
An interactive visual display is provided for passenger information and entertainment. In this illustration the passenger is shown in a somewhat sat-up position. It is anticipated that personal seating adjustment will be available to control the degree to which the passenger is sat-up or led out. The extent of such control will be determined by the specific implementation, in particular the headroom whilst the capsule is in the horizontal orientation.
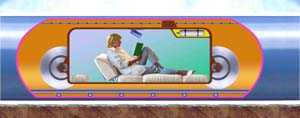
6. Performance
With the price of stations being relatively high (all the mechanics involved in acceleration, capsule handling and vacuum maintenance etc.), it may not be possible to provide so many stations as one would wish. However, capsule speed seems limited by very few factors, but probably the primary one is maximum acceptable acceleration for a human passenger. The design examples foresee a cruising speed in the order of 110 m/s for inter-city tubes, attained by acceleration at 5.5 m/s2 over a launch tube length of 1100 metres. A cruising speed of 110m/s equates to a Bristol (UK) to London journey time of just less than 30 minutes (120 miles~190km).
A launch tube length of 1100m provides certain disadvantages such as real-estate consumption and implications for the minimum distance between stations. For city-wide applications a much lower speed is anticipated in the design examples, being in the order of 30 m/s, again attained by acceleration at 5.5 m/s2 over a length of 82 metres.
The other speed-limiting factor is the minimum tube bend radius. In short, the straighter the tube, the faster the capsule/passenger can comfortably travel. Although, since the capsule velocity is a known constant, track camber can be used to keep the capsule safely on track. The presence of bends in the tube will also have implications for the operation of the motive power cord (refer Section 11).
For longer journeys such as US inter-state journeys some variations on the design will be necessary. Higher speeds are desirable to keep journey times comfortable, but in any case inter-state type journeys are clearly a long time in the future and could only ever be built after successful experience of smaller scale implementations.
The given inter-city design example provides for a twelve fold capacity benefit a traditional 6 lane highway with a consumed landscape benefit of better than five fold. Nonetheless, it should be mentioned that at least seven stations are required to feed an inter-city tube to capacity, and that theoretical maximum capacity is very unlikely to be attained in practice.
The most important benefit is that of energy consumption. A conservative estimate provides for at least a twelve fold benefit in the inter-city configuration and substantially more in the city wide configuration. Such a ratio provides for very substantial reductions in carbon dioxide emissions and related environmental concerns.
7. Advantages
The advantages of FTS include:-
Consumption of hydrocarbons reduced to almost negligible levels.
Carbon dioxide emissions reduced to almost negligible levels.
Significantly shorter journey times.
No traffic jams, congestion or unpredictable journey times.
Comparatively safe from human-error accidents.
Provides effective relief of congestion in other transport systems.
Vacuum tubes would consume only a faction of the landscape required
for rail or road systems and produce no discernible environmental or
noise pollution.
Departing passengers will travel when they are ready (not when the
train is ready, or according to a timetable).
Passengers will not need to stop at intermediate stations in which
they have no interest.
8. Disadvantages
The disadvantages of the concept are as follows:-
Significant capital investment to undertake feasibility studies,
design, development, trials and implementation.
Studies are required to assess the technical obstacles in station
design, departure acceleration and deceleration systems, motive power
delivery and safety systems.
Vacuum tubes will generally need to be straighter than the average
road.
9. Station Design
A station could appear much like an airport departure lounge, with capsule access doors along one side. On arrival at the station passengers will plug in their credit card to a check-in machine, key in their required destination code, go to the capsule access doors, step into the appointed capsule and the journey will begin. There will be a number of capsule access doors within the station to maximise the throughput of passengers. Behind the capsule access doors will be the vacuum area in which the capsules live. The capsule access doors will only open when a capsule is locked into position on the door. The capsule door and the station door will open together to maintain the vacuum.
Once the passenger (individually or perhaps as couples) enters the capsule it will re-orientate to the horizontal prior to departure, laying the passengers on their back for the most comfortable and efficient travelling position. Handling mechanics will relay the capsule to the launch tube. The launch tube runs parallel to the main tube and the capsule launch will be timed to coincide with a suitable gap in the flow of capsules.
Accelerating the capsules presents some significant technical obstacles, particularly since there will be a continuous flow of capsules entering the launch tube and the current design examples provide for up to 15 capsules in the launch tube at any one time. All capsules in the launch tube will be at different speeds and different stages in their acceleration profile. Delivery of acceleration force to capsules in the launch tube presents some of the most complex design issues.
Similar to the design issues of accelerating capsules, the reverse also applies in decelerating capsules at the destination station. As with acceleration, deceleration will usually be achieved in a separate deceleration tube. Although it may also be possible to design stations which accelerate and decelerate capsules within the main tube, the benefit being in cost savings.
10. Motive Power Cord Attachment
In principle it is a fairly simple matter to develop some mechanics permitting the capsule to attach to a motive power cord, since the capsule will already be travelling at the correct speed. In practice, the issue will not be so simple, as reliability is paramount since failure to attach will have serious consequences for the system. In addition there is the further complication in that the capsule will need to detach and re-attach to transfer from one motive power cord loop to the next. This mechanism will certainly be one of the major design issues.
11. Bends, Inclines & Freewheeling
At first sight one might assume that FTS will handle bends just as effectively as for example a roller coaster might. As with a roller coaster, track camber will be used to stabilise the capsule through bends.
Having established momentum and in the absence of air resistance, a capsule would theoretically maintain it's velocity even without the presence of motive forces, save for the effects of friction and inclines.
In practice, maintenance of a single motive power cord over the entire length of a tube might present unnecessary difficulties. One would wish to avoid so much as possible, the need for mechanics to enable the motive power cord to navigate bends in the tube. Moving parts such as idler wheels need lubrication, maintenance, cause friction, and provide for reliability compromises. The motive power cord will be present for the majority of the tube length, but for the remainder it's clear that capsules will freewheel for some period without loosing velocity to any detectable extent.
The motive power cord will exist in a number of loops, each loop generally covering a straight section of vacuum tube (running up in one direction and back again in the opposite tube). It will not generally be present through bends.
Capsules will detach the motive power cord at the end of the loop, freewheel for a short period and reattach to the next motive power cord loop when available.
12. Capsule Routing Mechanics
Traditional train wheels are designed with a shoulder to locate on the inside of the rail. Route switching is achieved by lateral track derailing mechanics. In short, the track is prepared to route the train off to the left or right and then prepared again for the following train.
There are two disadvantages with this approach for the FTS system. For the inter-city design example of 20 capsules per second, a time of only 50 mS exists from one capsule to the next. A time frame in this order is very short to achieve reliable track switching between capsules. The second problem is that of the 'easement factor'. A passenger involved in lateral acceleration (a quick jolt to the left or right) is far less comfortable than one involved vertical acceleration. In addition the incline of departure away from the main track should be kept very gradual and may take many capsule lengths to complete a full departure to a separate launch tube.
The proposed solution is as follows. Imagine that the rails are on a gauge of 1.7m as will be the wheel-base of the capsule. Prior to the point of interchange a second set of rails will begin running on a slightly wider gauge, say 1.9m, next to and on the outside of the main rails. The outer set of rails will depart from the main track by deviating slowly away vertically upwards. Capsules not destined to depart will retain their 1.7m wheel-base and follow through the junction between the deviating outer rails, without interference (no part of the capsule will overhang the wheels). Capsules destined to depart will widen their wheel-base to 1.9m at the appropriate time on instruction from the tube system. Such instruction could come electrically or mechanically. With a wide wheel-base the capsule will take the outer track/rails and divert up vertically away from the main track into the deceleration tube. For the system to function a suitably designed wheel profile would be necessary and the outer and inner rails need meet with a suitable connection as appropriate. The mechanics necessary to modify the capsule wheel-base would not be prohibitively complex or unreliable.
13. Passenger Comfort and Services
Since passengers will enter the capsules whilst they are vertical, it should not present more of a psychological problem than entering a lift (US: elevator). There is benefit in having reasonably small capsules and in having them led horizontally. The benefit comes in reducing the tube dimensions (and therefore construction costs). The amount of personal space around the passenger is a parameter to be determined in the final design. It would be unlikely to be less than available in an average family saloon car (US: compact). It would also be likely that the passenger has controls to permit sitting up to some extent, perhaps sufficient to facilitate reading a newspaper.
It must be anticipated that a small minority of passengers will suffer claustrophobia and anxiety from the acceleration and enclosed environment. One imagines difficulty with certain types of passengers, like the elderly. However there should be little real reason for anxiety with FTS than there is today with aircraft travel (after all people willingly travel thousands of feet above ground level).
With modern technology it will not be difficult to provide some passenger information in the form of an LCD visual display, indicating journey time or audio/visual entertainment etc.
Since capsule size is a construction cost issue, baggage could present some problems. The solution is for the automatic control system to allow the passenger a second capsule where large amounts of baggage are required. The automatic control system will keep both capsules together and deliver them to adjacent exit doors.
FTS will facilitate considerably shorter journey times and most passengers will not want personal services (food, toilet etc.). Where such services are required, the passenger will press a button within the capsule which will cause the automatic control system to route the capsule off the system at the next available station on route. The passenger will then make use of the station services and return to complete the journey in another capsule.
Should an urgent medical condition arise, again the passenger will press a button and likewise the capsule will exit at the next station. To save time it might also be possible for the automatic control system to alert medical services directly when the button is pressed.
Capsules will not require heating or be affected by cold whether since they remain isolated from prevailing whether conditions by the vacuum. It could be the case that over a long journey passenger body heat causes a rise in capsule temperature. Some capsule cooling mechanism might be necessary.
Passenger air supply will be contained within the capsule and recharged as necessary when the capsule is at rest in a station. The passenger will not generally be aware of travelling in an artificial climate controlled atmosphere. Emergency oxygen/air masks will be available to cover in the event of failure.
In general the public lacks enthusiasm for the restrictions imposed by safety belts/harnesses during travel. However, some form of restraint (perhaps voluntary) might well prove to be beneficial. The passenger will experience forces in one direction (head to toe or toe to head depending on design) during acceleration. Obviously the reverse direction will apply during deceleration. Clearly the passenger will feel more comfortable when the force direction matches that from gravity experienced walking the planet. In the reverse direction some arrangement is necessary to avoid the passenger going head long into one end of the capsule. Foreseen is some form of cushioned restraining levers/arms in a pair supporting the passenger around the shoulders such that acceleration forces are taken into the body through the shoulders/chest area. The passenger will pull such a restraint mechanism into place at launch time on a ratchet type arrangement, providing for a reasonably tight and secure restraint regardless of passenger size. The proposed acceleration employed in the computation examples is not excessive and will not cause general passenger distress.
Typically, the highest acceleration permissible for safety and comfort will be adopted. However the affect of da/dt (sometimes called the 'jerk factor') is equally as important as the absolute acceleration value. Whilst the human body readily accepts a given sustained acceleration rate (the unnoticed and everyday effect of gravity is almost twice that proposed for FTS), a sudden change on the other hand, leaves the muscles unprepared to stabilise and compensate the body. The value of da/dt needs to be limited by an acceleration profile which provides a lead-in and lead-out curve such that da/dt remains acceptable. Failure to do so would leave the passenger feeling an unwelcome shock effect at entry and exit of the acceleration phase.
The calculation examples provide for a rate of acceleration which is unlikely to cause significant blood rush towards the feet. Should such blood flow occur then temporary dizziness might result. In the final design it may be that public preferences determine the passenger position to be more sat-up to some extent. For example, reading a newspaper is not possible in the laid-out position but would be possible when sat as if seated in a canoe.
Where groups or families wish to travel together more than one capsule will be necessary. The automatic control system will provide for routing the capsules together and delivering them to adjacent exit doors. During transit communication between the capsules will be provided by use of the proposed interactive visual display units.
14. City-wide versus Inter-city Application: Examples
Computations have been undertaken for two different theoretical applications of the FTS concept. The first being a typical inter-city application and the second a city-wide application. The inter-city example employs parameters thought to be most applicable for a system aimed at medium range journeys, of say 15 to 400 km (10 to 250 miles). A trade-off exists between capsule speed on the one hand and construction and operating costs on the other (faster speeds requires straighter tubes and more power consumption in capsule acceleration).
In principle there is no reason why journeys of thousands of kilometres cannot be undertaken using the inter-city model, but typically passengers are bound to prefer journey times taking not much more than an hour. For this reason long haul or inter-state type journeys might employ higher speeds than the proposed inter-city example.
Whatever speed is chosen, it will never be appropriate for FTS to cross an earthquake fault line, or for example, to cross the Atlantic Ocean. In the city context, the operating speed of FTS is less important as journey times will in any case be very short. A lower speed is therefore proposed as it brings cost and flexibility benefits. When one considers the traditional car journey around a city, the need to stop at each traffic junction rapidly brings delays, unpredictability and congestion which is all to familiar. The FTS concept employs an automatic control system which has already planned the journey at its outset and allocated all necessary time slots and tube availability. To draw a parallel, its like being able to drive at full speed through all junctions with no need to check for impeding traffic.
In the city context, journey times are so fast, it may be the case that FTS can be constructed with only one tube and only one direction of travel, but in a loop around the city. In such a case, passengers travelling against the direction will make a complete loop around the city. A single loop will reduce construction cost considerably which will be very important in a city-wide context (some of the construction may need to be tunnelled). Of course a loop is not necessarily the optimum configuration for any given city.
15. FTS in a Public Context
Crime, vandalism and anti-social behaviour are synonymous with places like train and bus stations. Within the UK, for example, a police force has been created with specific jurisdiction for rail territory. The FTS concept has been designed for minimum staffing and fully automated operation. An FTS station will represent a large investment with significant high technology and safety critical components. Running video surveillance will be necessary to defend the station, but the capsule will be open to graffiti and similar abuse. To avoid such damage the following measures are envisaged.
Passengers using the system will be required to pay by credit card, or set up and an account before travelling. In this manner each passenger is traceable. Each passenger using a capsule will be required by terms and conditions to notify any damage or servicing need for the capsule, using the interactive visual display within the capsule. Failure to do so will make the passenger liable. The previous user of each capsule will always be known to the automatic control system and in this way any anti-social behaviour will be immediately traceable to the culprit, whose credit card will remain chargeable under the terms and conditions.
Forcing payment by credit card or account is also useful for other reasons. For example, the time taken by the passenger to enter and exit the capsule (the time whilst the capsule door is open) is important to system efficiency and throughput. It may advantageous to premium charge the passenger for excessive capsule entry/exit time.
Additionally, the system could hold in custody users of stolen credit cards, or those for which an arrest warrant exists, and report automatically to Police.
Prevalent in current transportation thinking in the UK is a concept known as an integrated transport policy. In short, improving the ability to link together disparate transport systems and provide a whole public transport system aiming closer towards a door-to-door solution.
Clearly FTS will never, in itself, provide a complete door-to-door transport solution for all of the population at all of the time. At best FTS could provide local stations in major urban areas and town by town stations in more rural and sub-urban zones.
However, FTS will have major advantages over rail travel in integrating with other forms of transport such as local taxis and buses. Since FTS has a much higher capacity than rail travel the economic motivation for other transport systems to link to FTS will be that much more pressing. In addition, trains deliver passengers one train load at a time, and of course the first passengers leaving the train take the taxis, whilst the remaining passengers must wait. Both passengers and taxis are left waiting for each other in a very inefficient manner. With FTS passengers will leave stations in a continuous stream, avoiding such a problem.
The technology used with FTS could also extend to integrating with local taxis. When the FTS passenger first enters a credit card and selects the destination the opportunity will exist to auto book a taxi and charge the entire journey in one commercial transaction. At the appropriate point in the journey the automatic control system will book the taxi by a direct link to a local taxi company and confirm the taxi arrival back to the passenger via the interactive visual display unit within the capsule.
If the passenger is requested for the final destination post code (US: zip code) then the taxi company could also be made aware at booking time providing the possibility of some further efficiency benefits. Likewise, before leaving home the passenger could book his journey by phone using a pre-programmed tone dial key sequence. For example, a single button press, pre-programmed for an FTS trip to the airport, should result in a taxi arriving at the door ten minutes later.
It's likely that many commuter type passengers will drive their cars to the station, take the FTS to work and return to their cars at the end of the day. Using the interactive visual display the automatic control system could query such passengers and propose car swap arrangements. In other words the passenger is proposed to loan his car to a fellow passenger whose destination station matches the users departure station. Financial compensation could be handled through the FTS passenger charging system.
Such an arrangement typically sounds very unpalatable to many car owners and its success might be questionable. However, such a system is environmentally and practically very sound, it frees up parking space, it gives the arriving passenger the freedom of car transport at the destination and would encourage use of FTS where otherwise the entire journey might be undertaken by car. Financial incentives could be used for encouragement or some kind of car-swap club.
The interactive visual display unit should play a useful role in making the journey more relaxing and enjoyable than present day commuting. Features such as interaction between passengers, audio/video entertainment and communications. The interactive visual display unit could also be used for reporting property left within capsules.
16. Freight
FTS is designed to address the urgent passenger transport issues of the day, without compromising in order to widen the scope of the system. However, given that a capsule can be dispatched directly to a specific destination, the system will also have many freight uses. However, the system will only be suitable for freight of a modest size (i.e. not bigger or heavier that a large man or couple).
The automatic control system will be able to assign capsule security codes to provide for collection only by authorised persons. One could imagine the sender of goods being given a security code when the goods are shipped (or perhaps choose his own code, or be given one in advance) which can be relayed to the receiver to ensure the correct recipient.
Capsule weight will be an important factor in making the acceleration mechanics work correctly. For that reason a maximum capsule weight will be necessary. An overloaded capsule (freight or otherwise), will automatically be prohibited from departing.
Freight pallets and shipping containers are outside of the capabilities of FTS. However, it could be the case that freight handling Companies have their own or specific access points into the network for freight use.
17. Financial
Cost/benefit analysis, projected investment returns, grant applications, construction costs, operational costs and computations for likely cost of travel are currently being researched and are under discussion with consultant engineers. It is intended to develop a pilot/demonstrator project in advance of a full implementation.
18. Safety
The safety record of road transport provides a fairly low benchmark against which FTS will be compared. The system will be safer than road transport in many ways, particularly driver related problems (tiredness, speeding, tailgating etc.).
FTS will also be safer than both road and rail in terms of environmental safety factors like whether (precipitation, ice, fog, flooding etc.) and obstacles (animals, branches, debris and the like). The system will also be safer in terms of accident causing mechanical failures (tyre blowouts, brake failures etc.).
However, when failures do happen the consequences will be very serious, more akin to air accidents. Unlike inter-continental air traffic, FTS does always provide the possibility of emergency services at least being able to get reasonably close to the injured to begin the process of extraction immediately.
Clearly safety is a very serious issue, but no more so that it already is with air traffic. Provided the safety issue is taken seriously FTS will prove to be one of the safest modes of transport available.
18.1. Derailment/Tube Damage/Obstruction
Should capsule derailment happen, the consequences will be serious. In effect a stream of capsules heading at very high speed towards a pile-up. Much like a highway pile-up. Usually capsules will travel very closely together. They will in effect all come to rest together, driving against the obstruction. A completely immovable obstruction is unlikely as the force of flowing capsules will be substantial and will generally push any obstruction on up the tube. It is not necessarily the case that all passengers involved will be fatally injured.
Clearly the objective must be to prevent the derailment in the first place by adopting safe design and a careful maintenance schedule with a statutory inspectorate.
The automatic control system will detect obstruction by verifying correct capsule movement using proximity detectors or the like, installed along the way. One would not wish the automatic control system to enter emergency shutdown routine on the failure, for example, of a single proximity detector. For this reason, addition verification might come from motive power cord tension, drive motor load current, and vacuum pressure detection.
Systems will be in place to detect and account for derailment of a capsule or a similar obstacle in the tube. On detection the system will enter a shut down routine immediately. It may be the case that the automatic control system has nowhere to route approaching capsules and contingency provision will be a significant design issue.
18.2. Bringing Capsules to a Halt in an Emergency
In an emergency capsules must be brought to a halt quickly. This can be done by two factors; release of vacuum in such a way as to offer maximum resistance, and resistance from the motive power cord itself.
Controlled release of the vacuum is a particularly effective way of bringing approaching capsules to rest. Depending on the relative dimensions of the capsule against the internal dimensions of the tube the ability for air to pass a capsule can be quite small. A retarding force of anything up to 20 tonnes of air pressure could theoretically be applied in the face of approaching capsules (although much lower force would be desirable and would be employed in practice). Effectively capsules come to rest on a cushion of air.
A stream of capsules all travelling in unison are better to brought to rest as one entity. It will generally be the case that the motive power cord remains intact at least up to the point of obstruction or tube damage. Therefore reverse force through the motive power cord will be the best way to bring capsules to rest. However, the possibility exists for the creation of emergency braking mechanisms within the tube itself. For example a braking surface such as a brake fibre strip (continuous or in segments) to effect friction between the tube wall and capsule stream at a suitable surface on the capsule.
Bringing to rest a stream of capsules at high speed will take a finite time. During such time the capsules at the head of the stream are being crushed. However, this will only happen in the event of a total blockage sufficient to completely resist the force of approaching capsules. Such a blockage is very unlikely but should it happen the effect might be alleviated by removal of a segment of the tube wall at the point of obstruction, or somewhere slightly in advance. In order to be effective the removal of such a segment would have to be automatic and immediate.
18.3. Emergency Escape
The tube could be manufactured from a number of substances, but the most obvious is pre-fabricated concrete sections. Such sections would need lining or impregnating with a suitable compound such as a resin for sealing purposes.
Evacuating passengers in an emergency will require the emergency services being able to break into the tube, either at will or at regular designated points along the way. The emergency services could be prepared in advance with mechanisms for delivering an explosive charge to pre-determined break-out panels delimited by fracture lines designed into the tube at manufacture.
As the automatic control system detects obstruction or loss of tube integrity, it will determine the need to release vacuum to atmosphere and the point at which such release should be made. The release of vacuum will be automatic and will happen rapidly following detection of the problem. Release of vacuum could be via removal of a break-out panel in the tube wall. Such removal could happen as a result of an explosive charge implanted at break-out panels along the way.
The mechanisms (explosive or otherwise) to release break-out panels could be permanently available at strategic points along the tube and under the control of the automatic control system. Permanently resident explosive charges are not so implicitly dangerous as at first sight seems. They are for example present all modern motor vehicle air-bags.
Permanently available break-out panels might need certain scheduled maintenance, and of course carry the possibility of false detonation they may in reality prove themselves to be more dangerous than helpful.
The extraction of large numbers of passengers from a long and possibly underground tube in an emergency situation is a very important consideration. Additional systems for emergency extraction of passengers could be warranted. Such systems could be constructed in a number of ways. For example, a specifically designed and remotely controlled small mechanical unit could be constructed to drive along the track underneath a line of capsules needing to be extracted. The unit would tow a steel wire and attach it at the front of a group of capsules providing the possibility of drawing the capsules back up the tube, thus facilitating escape.
Air-bag technology could also be feasible with FTS. The possibility is for the deployed air-bag to be large enough to virtually fill part of the capsule, thereby pinning the passenger(s) against the cushioned bed surface below in a secure, safe and tightly restricted position.
Oxygen/air supply following air-bag deployment could present some particular difficulties. It may also be the case that deployment of such a large air-bag will take more time than the motor vehicle case, but in reality the deployment may need to be faster given the higher speeds involved. Deployment of a very large air-bag will result in a sudden change of air pressure which could in itself have some uncomfortable consequences. Further research is required in this area.
18.4. Capsule Strength and Integrity
The capsule must be capable of retaining a pressure of one atmosphere (about half the pressure typically used within a car tyre). Should the capsule pressure seal be broken, in the event of an accident or for whatever reason, loss of pressure is likely to cause passenger injury in the form of internal bleeding etc. The presence of an emergency oxygen/air mask will not help against sudden pressure loss. Capsule strength must therefore be sufficient to minimise such injury. Research to maximise the ability of the capsule to retain integrity in all circumstances is imperative.
18.5. Terrorism
Unfortunately FTS will present the ideal terrorist target. Bombing of any one tube section will cause both loss of life and bring the entire system rapidly into difficulties. Defence of the system being quite difficult due to the area involved.
18.6. Fire
Fire cannot happen within the tube. Nothing flammable will exist within the capsule except anything carried by the passenger. Fire within an individual capsule will be detected and extinguished by a suitable means. The capsule will be routed to the nearest exit. The passenger will have an emergency oxygen/air mask available.
18.7. Station Behaviour in Systems Failure Mode
The automatic control system will manage all aspects of the system under
normal conditions. On failure all elements of the system must fail to
the safest state. For example where a station has lost communications or
is aware of a need to enter emergency mode it will behave in the
following fashion:
No more passengers will be permitted to enter the system.
Those departing capsules not already committed to launch will be
returned to the entry/exit doors to eject passengers.
The station will employ all entry/exit doors for removal of
passengers.
The station will employ its full capacity to draw capsules from the
main tubes regardless of the intended destination of the passengers.
19. Maintenance
With the consequences of failure being serious, maintenance takes a high priority. Obviously passengers will want the system 24 hours/day, 365 days/year, but the system will need to be shut down for maintenance. What is crucial is that passengers know, understand and accept when the system will be shut down. For example, the system is always and only shut down between 10:00pm on Sunday until 06:30am Monday morning.
Tube and track maintenance will present some new engineering problems. For example, it is the case that standard grease packed bearings will not operate well in a vacuum environment. Wherever, grease or oil exists in the absence of air pressure, rapid evaporation occurs since the liquid state of such substances is generally less sustainable as pressure decreases. Although the vacuum adds some problems it also removes some others. For example, rust, lichen, moss and erosion do not feature in a vacuum.
Obviously telemetry will be used to monitor maintenance issues such as motor failures etc. However, much of the mechanics will be duplicated for reliability reasons. For example, all drive motors will be duplicated, such that motor failure does not cause system failure. It may be the case that drive motors can exist on the air side rather than the vacuum side, but obviously the air-seal issue presents itself.
Since it is never planned to release the vacuum, space suits will be needed for some manual maintenance of vacuum side mechanics. The design will aim to minimise the effort involved in a number of ways. A manned maintenance capsule will exist which can navigate the tube system during a shut-down maintenance period. Where maintenance needs are expected the mechanical units will be manufactured in such a way as to provide plug-in replacement where possible. The replacement of such units will be possible through use of a maintenance capsule. To take an example, should a motor failure happen or a service be required, the maintenance capsule would be used to replace the motor and retrieve the original for service.
It should be remembered that electric motors employed for tube motive power will be much smaller than those required for capsule acceleration. Ultimately it may even be possible to replace track segments with the use of maintenance capsules.
Of course it will be the case that much of the mechanics cannot be plug- in replaced in that way. Consideration will be given to the development of some method of plugging the tube either side of a site needing maintenance whilst releasing the vacuum at the maintenance site itself.
What might be possible is a form of plug which latches against the perimeter of the tube. However such a plug would need to sustain a pressure of up to 20 tonnes if the internal cross sectional surface area were say 2m2 (and it's likely to be more).
Individual capsule maintenance does not present a serious problem. Each capsule will be uniquely numbered with its own maintenance schedule and history. Capsules can be taken from the tube system at a specially designed capsule removal point, within a maintenance area at one specific station. Capsule maintenance can take place without affecting on going operations.
Small amounts of air leakage are expected at the capsule access doors, but maintenance of the vacuum should not present significant problems. Whilst an absolute vacuum is unnecessary, a certain level of vacuum will need to be constantly maintained by one or more vacuum pumps around the system.
20. Environmental
From an environmental view point FTS delivers results that far exceed anything currently available. Whilst there are many environmental good ideas, like solar cars and catalytic converters, none of these has the potential to even begin to make any real difference to the global problems of the day. FTS can; motorists will convert as the congested highways simply cannot compete on the one thing that matters: speed.
Relief of congestion is not the only environmental benefit. The absence of air resistance, coupled with the delivery of motive power on a system wide basis will bring significant and real benefits in the consumption of hydro-carbons. Further, since motive power will be generated by a small number of large and powerful electric motors, pollution, noise and emissions are not really relevant to FTS.
Another fuel consumption benefit of FTS is that capsules will all travel at the same speed. There will be no wasted energy due to acceleration and braking. Moreover, the energy consumed to climb hills might also be retained instead of wasted, if the motive power cord can be arranged to transfer the downhill excess of energy from one capsule into up hill energy for another (this would be a detailed design issue). It may also be possible to avoid loss of energy due to capsule deceleration at destination and recycle this energy back into the system (again this would be a detailed design issue).
Noise pollution will be minimal as the capsules will travel within a vacuum and noise cannot transcend a vacuum. Environmental damage due to the presence of tubes across the countryside, is an issue, but the tubes will take considerably less space than the alternatives, and might perhaps be laid underground in some places. Moreover, the FTS tubes will be substantially less intrusive to flora and fauna than would traditional road or rail.
21. Road Traffic Comparisons
For the purpose of this document comparisons are made of car against capsule rather than passenger against passenger. Subject to the ultimate design, FTS capsules might allow for single persons, or more likely the design will allow for pairs/couples as well. Since in practice the vast majority of cars also contain either one or two passengers the comparison appears broadly valid. Comparison of FTS versus a 6 lane highway:-
Real-estate: 5.4:1 (i.e., a better than five fold advantage,
FTS versus 6 lane highway, note 1)
Station Capacity: 5.4:1 (One station versus a single carriageway;
2000/hr)
Tube Capacity: 12:1 (Tube versus six lane highway, note 2)
Energy Consumption: > 12:1; 13.7:1 = 23 x 103 kW / 1684 kW (inter-city, note 3); 168:1 = 23 x 103 kW / 137 kW (city-wide, note 4)
Congestion: N/A (note 5)
Airborne Pollution: N/A (note 6)
Noise: N/A (note 7)
Note 1: In this example FTS tube is foreseen to be 2.3m wide, resulting in 4.6m per tube pair. A six lane highway is at least 25m wide.
Note 2: For the inter-city example, FTS will provide for 20 capsules per second as against the figure of 2000 cars per highway lane per hour which equates to approximately 0.6 cars/second.
Note 3: The 13 fold energy advantage shown here is taken from the inter-city 2000 passengers per hour scenario on a typical 15km journey. These figures come from computation shown appendix A.
Note 4: The 168 fold energy advantage shown here is taken from the city-wide 2000 passengers per hour scenario on a typical 15km journey. These figures come from computation shown appendix A.
Note 5: FTS will not suffer congestion as the automatic control system will manage capsule traffic on a system wide basis, and route, destination capacity and tube availability will all be established prior to capsule departure.
Note 6: FTS will create no air-borne pollution, save for indirectly at power stations as a result of electrical energy consumption.
Note 7: Since noise cannot transcend a vacuum the only noise generated by FTS will be from the driving electric motors at the station. Any noise generated by travelling capsules will be such noise as can be conducted through the track and then radiated on through the bulk and fabric of the tube itself. The noise thereby generated is though to be negligible.
22. Social/Economic Impact
A full-scale network implementation of FTS in, for example the UK, would bring the bulk of the population of England within commuting distance of the capital and conversely the regions within more easy reach of the majority population. Substantial social/economic impact could result.
Even perhaps so far as some levelling of real-estate values and maybe even some potential for re-invigorating the more deprived and far flung areas.
Environmental impact, cost benefit analysis and social/economic impact assessment are all important areas of study necessary to argue the case for or against the FTS system. However such issues are outside the scope of this document.
Appendix A - Concept Validation Calculations
A.1 Capsule Velocity
Affecting Factors:
All capsules will travel in unison at a fixed speed. Higher speeds bring benefits in system passenger capacity and lower journey times. Lower speeds bring benefits in terms of the ability of the system to tolerate a less straight tube network, and shorter launch tubes. Whereas it's important to reduce journey times with inter-city applications, city-wide applications require short launch tubes. Different operational speeds are proposed for these differing applications.
Computations:
30m/s being the computations example for city-wide applications and 110m/s being the example for inter-city. These figures provide for realistic lengths of launch tubes (refer to acceleration section) and convert to 108km/h and 400km/h respectively (67 & 250mph).
A.2 Capsule Acceleration
Affecting Factors:
Higher acceleration/deceleration rates provide for shorter launch tubes, which may help in the reduction of consumed real estate. However it may also likely be the case that, in the final design, launch tubes run above or below the main tubes and therefore no real-estate advantage. Very long launch tubes could limit the location of stations, as they must of course be sufficiently far apart for the distance to be at least twice the length of a launch tube. Lower acceleration / deceleration rates provide better passenger comfort. Although it's not a major effect the capsule velocity on entry to the launch tube does effect the launch tube length.
Typically, the highest acceleration permissible for safety and comfort will be adopted.
Computations:
The proposed figure for acceleration of 5.5m/s2 is less than available with a sports car and equates to a little over ½ g. This figure is thought to be reasonable and acceptable for the general travelling public. This acceleration figure is around 4 times that employed in passenger tram systems (typically 1.4m/s2), but clearly horizontal and restrained passengers will tolerate substantially higher acceleration than free standing passengers.
At the proposed inter-city speed of 110m/s with a launch tube entry speed of 3m/s a launch tube length of 1100m is required (and similarly required for final deceleration).
Inter-city:
Vf2 = Vi2 + 2as
1102 = 32 + 2 x 5.5s therefore s = 1100m = Launch tube length
Over a period of (110-3)/5.5 = 19.5s = Launch time
City-wide:
Vf2 = Vi2 + 2as
302 = 32 + 2 x 5.5s therefore s = 83m = Launch tube length
Over a period of (30-3)/5.5 = 4.9s = Launch time
Max capsules concurrently in launch tube,
given say a launch tube entry velocity of say 3m/s
a capsule length of 3.5m with gap of 0.5m
Each capsule takes (3.5+0.5)/3 = 1.333 seconds to enter launch
Inter-city:
Launch time = 19.5s
Max capsules in launch tube = 19.5 / 1.333 = 15 capsules
City-wide:
Launch time = 4.9s
Max capsules in launch tube = 4.9 / 1.333 = 4 capsules
Notes:
For the purposes of acceleration computations the figures used are the
effective acceleration over the complete profile including lead-in and
lead-out curves required for da/dt ('jerk factor') limitation purposes.
A.3 Mainline Vacuum Tube Capacity
Affecting Factors:
Higher capacity is gained by reducing inter-capsule gap and/or increasing capsule velocity. The minimum inter-capsule gap could be very small, perhaps a matter of inches, save for the fact that capsule routing mechanics will require a finite switching time and must be able to switch states between two capsules.
Computations:
Taking say, 2m as a minimum inter capsule gap and 3.5m for the capsule
length, tube capacity is as follows:-
Max tube capacity = velocity / (capsule length + min inter capsule
gap)
Max inter-city capacity = 110 ms / (3.5m + 2m) = 20 caps/s
Max city-wide capacity = 30 ms / (3.5m + 2m) = 5.45 caps/s
A.4 Station Capacity
Affecting Factors:
Station capacity is limited by the number of passenger access doors (entry/exit doors), the speed at which passengers use the access doors, the ability of the mechanics to handle capsule throughput, and the capsule velocity at the point of entry/exit to the launch tubes.
Obviously the inter-capsule gap will increase with velocity whilst the capsule rides in the launch tube (and conversely decrease with deceleration). This effect can be employed to funnel launch tubes for higher capacity. In many stations funnelling will not be necessary. However, given say a 50 access door station, a four into one funnelling will provide more than sufficient launch tube capacity even in the case where all access doors are employed for departure and all passengers departing in the same direction.
Computations:
Given say:
Launch tube entry velocity of 3m/s
Capsule length of 3.5m
Inter-capsule gap at launch tube entry/exit 0.5m
Maximum 'access doors open' time of 10s
Capsule access door vacuum locking time of 4s
Launch tube funnelling of 4:1
50 capsule access doors per station
Station throughput based on launch tube capacity:
3m/s with 3.5m capsules and 0.5m gap = 0.75caps/s
4:1 funnelling provides for 3caps/s
Station throughput based on passenger access doors:
Maximum passenger entries per door = 60s / (10s + 4s) =
4.286caps/minute
Maximum passenger throughput = (4.286 x 50) / 60 = 3.57caps/s
Notes:
Given a station capacity of 3caps/s and an inter-city tube capacity of 20caps/s some seven stations are required to feed one inter-city tube to the maximum capacity.
A.5 Energy Consumption
FTS Consumes energy in the following ways:-
Energy to accelerate capsules.
Energy to overcome bearing frictions.
Energy to drive capsule handling mechanics.
Energy to facilitate capsule ascent of inclines.
Energy to maintain vacuum.
FTS might recover energy in the following ways:-
Energy to decelerate capsules.
Energy resulting from capsule decent of inclines.
Whilst energy resulting from decent of inclines is logically and more easily recovered back into the system, the energy resulting from capsule deceleration might either be wasted to atmosphere or recovered back by employment of suitable mechanics. Additionally, with FTS acceleration becomes a one off expenditure of energy, regardless of journey length, rather than recurring cycle of energy expenditure as is the case for motor vehicles.
Many differing approaches can be made to forecast the energy consumed and what percentage might be recoverable. Whilst friction from bearing efficiencies may not be negligible, the specific computation will ultimately depend on many design specifics.
Considering acceleration alone. A typical motor vehicle journey might consist of at least dozen stages of acceleration, on the other hand FTS has only one. Broadly then, FTS benefits from a twelve fold advantage.
Considering bearing efficiencies alone. Within a motor vehicle bearing surfaces exist in the wheels, axles, differential, gearbox, clutch and engine (friction losses extending still further with automatic transmissions). It's not at all difficult to count at least a dozen occurrences of bearing surfaces within the typical motor vehicle for every one employed with an FTS capsule.
The complexities of considering friction losses extend still further when considering system utilisation levels. A fair percentage of the friction losses with FTS exist, regardless of utilisation levels, whereas friction losses are proportional to utilisation with motor vehicle travel. Suffice to say that, whilst friction losses may be a small but significant proportion of energy consumed by FTS, it is generally going to be significantly less than that consumed by a motor vehicle. And in any case bearing efficiencies within a motor vehicle constitute a small fraction of consumed energy when compared to air displacement.
Whilst air displacement constitutes a large proportion of consumed energy with motor vehicle transport, the loss is virtually nil with FTS. On the other hand FTS must maintain it's vacuum and some constant pumping is likely to be necessary. However, the feeling is that the energy consumed by FTS in maintenance of vacuum for the entire system is probably less than the energy consumed by one or two vehicles. In this case FTS is bound to have a much better than twelve fold advantage.
One can readily obtain rough estimates for energy consumption in the case of a motor vehicle, with FTS the calculation very quickly becomes academic and hypothetical. In the motor vehicle instance, any given motor car engine might generate say 40 or 50kW in operation. Such energy displays itself in engine heat, brake disk heat, exhaust fumes heat, acceleration, air displacement, accent of inclines, and friction losses.
For FTS the answer will not be known until a pilot project has been constructed. However, particular experimentation has been undertaken to establish the order of magnitude of energy consumed by friction in an FTS scenario. A 50mm diameter steel axle shaft has been mounted on two standard grease packed and sealed caged ball bearing races with a single wheel mounted on one end. A wheel fabricated from 3mm sheet mild steel and with a diameter of 762mm was employed. A rotational force at the radius was applied sufficient to break the frictional bond and commence movement. To counter any possible minor imbalances the experiment was repeated for rotation in both directions. The fact that breaking the frictional bond requires slightly more force than maintaining constant motion has been ignored for simplicity. The figures obtained are by no means definitive, but rather for concept proving and road traffic comparison purposes.
A 15 gram weight applied at the point of radius intersection with a horizontal line through the axle turns the wheel repeatably and in either direction.
Force = 15 x 10-3 x g = 0.14715 N (where g = 9.81)
Since the moment is at 381 mm factoring up for an FTS proposed 1m
diameter wheel:-
Force to draw capsule on friction of one wheel = 0.14715 N x 381mm /
500mm = 0.1121 N
Force to draw capsule on friction of four wheels = 0.1121 N x 4 = 0.4485
N
Power (inter-city) = F.v = 0.4485 N x 110 m/s = 49 watts.
Power (city-wide) = F.v = 0.4485 N x 30 m/s = 13 watts.
The power being consumed is somewhat similar to that being produced by a small electrical soldering iron (which would typically be around 25 watts). In reality the frictional power consumed is likely to be somewhat less than this experiment suggests as the experiment was undertaken with cold greased bearings and allows for four bearings per axle which may not be the case. Nonetheless there may be a potential problem in heat dissipation as all heat must be conducted into the capsule structure with no free air present.
The drawing force can also be derived by pure arithmetic by employing the
following relationship:-
M = f x P x Ci/2
Where: M is the torque resistance, P is the bearing load, F is the bearings coefficient of friction, Ci is the diameter of the inner raceway.
A typical value of f for radial caged roller bearings is 0.003 (e.g., Nadella Corp).
M = 0.003 x 500kg x g x 0.05m / 2 = 0.369Nm (where g=9.81)
Factoring up for a 1m diameter wheel gives:
F = M / radius = 0.369 / 0.5 = 0.738 N
Note: With use of this formula the number of bearings and/or wheel is irrelevant and cancels out as the weight divides between them.
Since the ways in which energy is consumed differs widely between FTS and traditional motor vehicles, many ways of making comparison are possible. The particular scenario chosen here is for a 15km journey by 2000 cars/capsule per hour.
FTS Energy consumption:-
Acceleration of 2000 capsules per hour:
Energy consumed to accelerate 1 capsule = ½ M (Vf2 - Vi2)
Taking the loaded capsule mass to be say 500kg:
Inter-city: ½ 500 x 1102 = 3,025 kJ over 20.5s = 150 kW (= 200 hp)
City-wide: ½ 500 x 312 = 240 kJ over 5.7s = 42 kW (= 56 hp)
Therefore to accelerate 2000 capsules in 1 hour consumes:-
Inter-city: Power = Work/Time = (3,025 kJ x 2000)/(1x60x60) = 1680 kW
City-wide: Power = Work/Time = (240 kJ x 2000)/(1x60x60) = 133 kW
Time in transit (ignoring acceleration/deceleration time):
Inter-city: 15 km / 110 m/s = 136 s
City-wide: 15 km / 30 m/s = 500 s
Therefore with 2000 capsules per hour, one capsule is launched every 1.8 seconds.
Number of capsule in transit at once:-
Inter-city: 136 / 1.8 = 75.5 capsules
City-wide: 500 / 1.8 = 278 capsules
Energy consumed by the system:-
Power = Acceleration power + Friction consumed power in transit
Inter-city: 1680 kW + (49 watts x 75.5 capsules) = 1684 kW
City-wide: 133 kW + (13 watts x 278 capsules) = 137 kW
(energy consumed by friction during acceleration/deceleration has been ignored).
Energy consumed in a motor vehicle scenario:-
If one assumes a 15km journey takes 20 minutes and each car consumes in
operation an average say 35 kW:-
Motor Vehicle Power = 35 kW x 667 cars = 23 x 103 kW
Whilst to some lesser extent, the advantages of FTS over car also exist to some extent in railway and coach transport. However the fact remains that the problems of speed, congestion, submission to a timetable and stopping at intermediate stations, mean that these forms of public transport will simply never deliver a convincing impact on the existing problems of the day.